Der Inhalt dieser Seite ist leider nur auf Englisch verfügbar.
New ALICE results show novel phenomena in proton collisions
2017-05-08 – News from the Physics Department
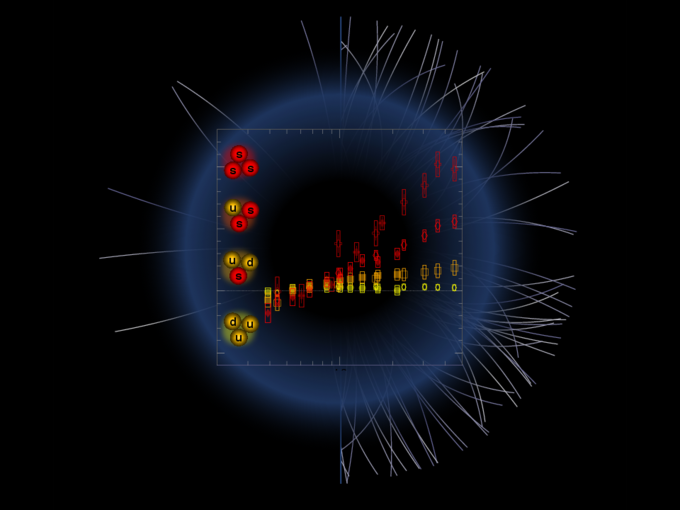
Strange hadrons are well-known particles with names such as Kaon, Lambda, Xi and Omega, all containing at least one so-called strange quark. The observed ‘enhanced production of strange particles’ is a familiar feature of quark-gluon plasma, a very hot and dense state of matter that existed just a few millionths of a second after the Big Bang, and is commonly created in collisions of heavy nuclei. But it is the first time ever that such a phenomenon is unambiguously observed in the rare proton collisions in which many particles are created. This result is likely to challenge existing theoretical models that do not predict an increase of strange particles in these events.
“We are very excited about this discovery,” said Federico Antinori, Spokesperson of the ALICE collaboration at the Large Hadron Collider (LHC) at CERN. “We are again learning a lot about this primordial state of matter. Being able to isolate the quark-gluon-plasma-like phenomena in a smaller and simpler system, such as the collision between two protons, opens up an entirely new dimension for the study of the properties of the fundamental state that our universe emerged from.”
The study of the quark-gluon plasma provides a way to investigate the properties of strong interaction, one of the four known fundamental forces, while enhanced strangeness production is a manifestation of this state of matter. The quark-gluon plasma is produced at sufficiently high temperature and energy density, when ordinary matter undergoes a transition to a phase in which quarks and gluons become ‘free’ and are thus no longer confined within hadrons. These conditions can be obtained at the Large Hadron Collider by colliding heavy nuclei at high energy. Strange quarks are heavier than the quarks composing normal matter, and typically harder to produce. But this changes in presence of the high energy density of the quark-gluon plasma, which rebalances the creation of strange quarks relative to non-strange ones. This phenomenon may now have been observed within proton collisions as well.
In particular, the new results show that the production rate of these strange hadrons increases with the ‘multiplicity’ – the number of particles produced in a given collision – faster than that of other particles generated in the same collision. While the structure of the proton does not include strange quarks, data also show that the higher the number of strange quarks contained in the induced hadron, the stronger is the increase of its production rate. No dependence on the collision energy or the mass of the generated particles is observed, demonstrating that the observed phenomenon is related to the strange quark content of the particles produced. Strangeness production is in practice determined by counting the number of strange particles produced in a given collision, and calculating the ratio of strange to non-strange particles.
Enhanced strangeness production had been suggested as a possible consequence of quark-gluon plasma formation since the early eighties, and discovered in collisions of nuclei in the nineties by experiments at CERN’s Super Proton Synchrotron. Another possible consequence of the quark gluon plasma formation is a spatial correlation of the final state particles, causing a distinct preferential alignment with the shape of a ridge. Following its detection in heavy-nuclei collisions, the ridge has also been seen in high-multiplicity proton collisions at the Large Hadron Collider, giving the first indication that proton collisions could present heavy-nuclei-like properties. Studying these processes more precisely will be key to better understand the microscopic mechanisms of the quark-gluon plasma and the collective behaviour of particles in small systems.
The ALICE experiment has been designed to study collisions of heavy nuclei. It also studies proton-proton collisions, which primarily provide reference data for the heavy-nuclei collisions. The reported measurements have been performed with 7 TeV proton collision data from LHC run 1.
The Fabbietti group at the Physics Department of TUM is primarily involved in the future hardware upgrade for the ALICE experiment as well as working on data readout and analysis. The main component of the ALICE experiment is the world’s largest so called TPC (time projection chamber), which records the tracks and the speed of fast charged particles. This detector covers an area of around 34 square meters and will be replaced by much faster technology developed at TUM.
Publication
Links
- Research group Fabbietti
- ALICE detector at CERN
- CERN (Conseil Européen pour la Recherche Nucléaire)
- Welt der Physik (in German): wie unmittelbar nach dem Urknall
- Universetoday: Another Strange Discovery From LHC That Nobody Understands
- Newsweek: Strange Quarks at Large Hadron Collider Shed Light on Universe’s ‘Primordial Soup’ Just After the Big Bang